Perseverance has landed
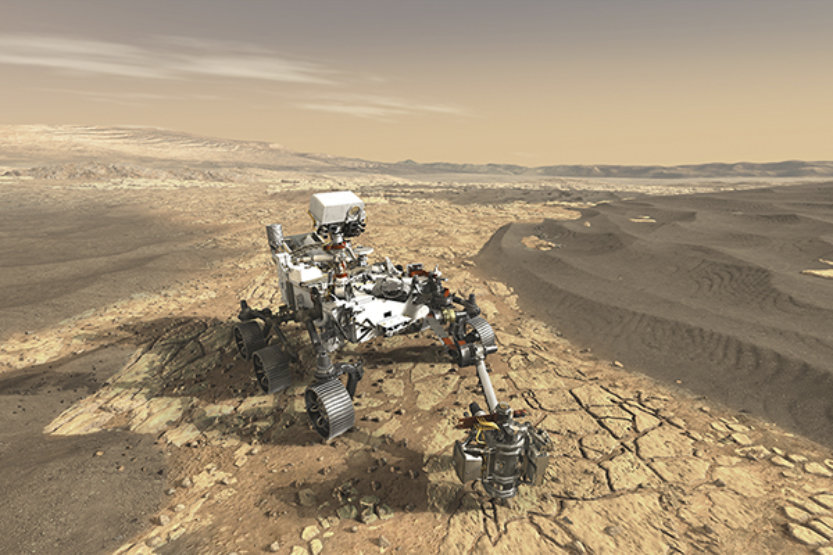
DATE: Feb. 18, 2021
PLACE: Outer atmosphere of Mars
Seven minutes of terror—that’s what people at NASA call atmospheric reentry, an interval of conditions so extreme that the spacecraft’s communications go silent. That’s where the capsule carrying the Perseverance rover is at the moment, having separated from the cruiser that has propelled it across time and space—seven months and 300 million miles—to the orbital field of Mars.
As it arrives on the cusp of the atmosphere of Mars, Perseverance has decelerated from its interplanetary cruising speed of Mach 18—approximately 13,339 miles per hour—to a mere 1,000 mph. The capsule deploys a parachute to slow its descent. It creates drag in the freefall-friendly Mars atmosphere, which is thinner than Earth’s by a factor of 100. The capsule plunges through the atmosphere, buffeted by turbulence. Flames as hot as the Sun’s consume its heat shield. Soon, the heat shield is ejected and the parachute cut loose, so that the jet pack carrying the rover can zoom free and head to the surface at about 70 mph, the same speed cars do on the interstate. It sets the rover down as though this was a construction site and not the pocked, stony, barren surface of the Red Planet.
Just seven minutes from the top of the Mars atmosphere to the NASA-selected site on the edge of Jezero crater.

Believed to be the remains of an ancient delta, Jezero Crater is an ideal location to explore for signs of fossilized microbial life on Mars. (ESA/DLR/FU-Berlin/NASA)
Worldwide, people sit at computers and watch TV screens, leaning into the long, blacked-out interval, as commentators describe what’s going on with Perseverance. Post-landing, they wait out the additional four-and-a-half minutes it takes for the Perseverance to reach back to Earth with the message: Safely landed and reporting for duty.
In the top percentile of those tuned into the landing is Zach Putnam, an aerospace engineer at Illinois, whose research has played a part in designing the Perseverance’s landing system. “It’s a little bit nuts,” says the genial Putnam about the unlikely steps required to go from hypersonic interplanetary travel to setting down a large object on Mars’ surface with what NASA calls a “sky crane.” But he points out that this landing, like the rover Curiosity’s landing before it, represents “a pretty well-defined progression from technology that was developed in the ’70s up until now.”
Putnam has spent his career in the tricky, high-stakes, immensely complicated study of reentry—a modest term for rapid descent from insane altitudes at mind-blowing speeds into hostile environments. He’s done a stint on NASA’s Project Orion, a spacecraft designed to carry astronauts to the Moon and into deep space and return them safely to Earth. His lab at the University of Illinois has won grant after grant from the space agency and other funding sources to support its work on how to safely and accurately land spacecraft on other planets.

Aerospace Engineering Professor Zach Putnam and his lab at Illinois work on reentry, the journey that takes a spacecraft from the outer atmosphere to the landing surface. Critical to this complex, high-stakes process is the supersonic parachute, such as the one deployed during the Feb. 18, 2021, landing of the Perseverance on Mars. (T. Wayne/NASA/JPL-Caltech; Inset: UI Public affairs)
Landing options for heavier spacecraft
It takes a mighty big parachute to make this happen. One that’s more than 60 feet in diameter and could, as Putnam observes, “cover your house.”
Parachutes have been proving themselves in space exploration since the first one floated astronaut Alan Shepard and his capsule safely down into the Atlantic Ocean on May 5, 1961, concluding America’s first manned space launch. In 2012, the Curiosity rover made it to Mars with essentially the same parachute (and essentially the same seven minutes of terror faced by Perseverance).
In June 2020, Putnam co-authored an article for the Journal of Spacecraft and Rockets that explained how to slow a spacecraft as it enters the Martian atmosphere. After the capsule detaches from the interplanetary cruise phase, “It turns out the best thing to do is to immediately dive deep into the atmosphere,” he explains. “When the spacecraft reaches an altitude where the atmosphere is relatively thick, it executes a pull-up maneuver with the force of its aerodynamic lift.” This maneuver allows it to fly horizontally at a lower altitude where there is more atmospheric density to slow the spacecraft and help the parachute deploy.
Looking to the future of Mars exploration, Putnam sees the need to lose the chute. “The weight of the rover is around 2,000 pounds—[equivalent to] a small car,” he says. “The next step would be to land something much larger—comparable to an SUV.” Doubling payload, however, exponentially increases the required parachute size.
As a spacecraft’s size gets larger, “It’s not long before you get a 500-meter-wide canopy,” Putnam says. “That’s big enough to cover Memorial Stadium.” The logistics of deploying such a beast become so complicated and dangerous as to render it infeasible. “It’s hard enough to get the parachutes we have to inflate,” he says. “A parachute that doesn’t deploy correctly just rips itself to pieces, and then the spacecraft is literally trailing ribbons. That doesn’t do much to slow it down.”
So how to skydive—or, rather, spacedive—without a parachute? The most obvious way would be by supersonic retropropulsion—firing the capsule’s rockets against the pull of gravity to slow and control its descent. That technology is already at the command of SpaceX. The aerospace company’s Falcon 9 rocket, which boosted its latest array of satellites into orbit last August, returned to settle, nose-up, on a platform floating in the Atlantic Ocean. It’s the sixth time the craft has gone into space and come back to Earth, ready to do it all over again.
A retropropulsion landing on the Red Planet creates a weight problem of its own, though. While the interplanetary journey that gets the spacecraft to Mars is solar-powered, a retropropulsion touchdown requires propellant and “propellant is heavy,” Putnam points out. “Every pound or kilogram of propellant you have to carry is one less pound or kilogram of astronauts or scientific instruments or food or water. It’s pretty much a one-to-one tradeoff. We like to use as little propellant as possible so that we have room for everything else we want to bring with us.”
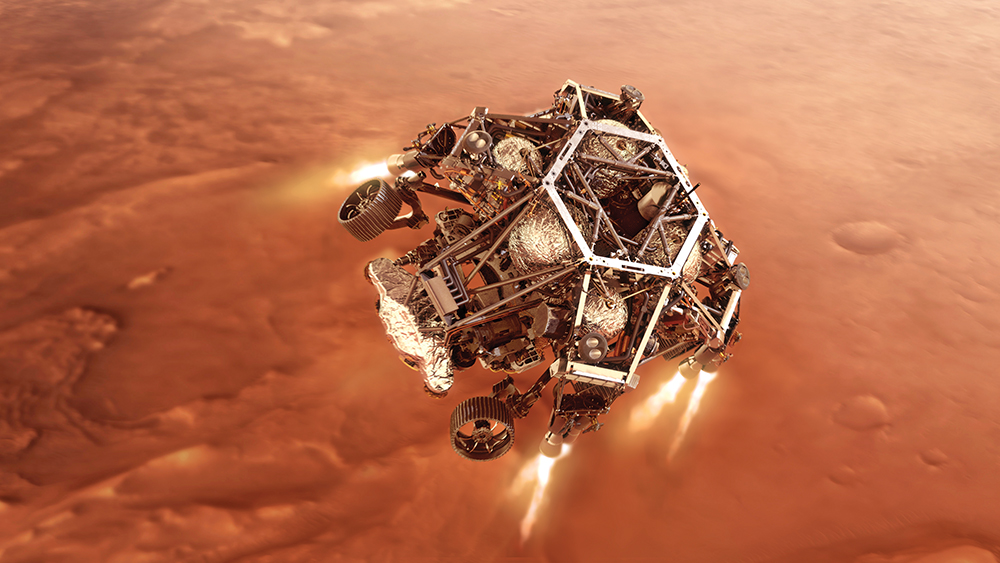
After the parachute is jettisoned, the Perseverance uses descent stage engines, which act as a jet pack to position the 2,000-pound rover and slow it to its landing speed of 70 mph. (NASA/JPL-Caltech)
Putnam’s research group works on optimal trajectories, which means determining the best techniques and flight paths for descent and landing. Current projects include experiments with non-parachute devices to create drag. “Imagine if you had a space capsule and around it was an umbrella kind of thing that you could pop out,” Putnam suggests. “And all of a sudden, instead of the vehicle being five meters in diameter, it would be 10 meters, giving it just a bit more mass.”
The group also is researching novel reentry trajectory control schemes. “We’ve been doing some work looking at flaps,” Putnam says. “Like the flaps on airplanes that pilots use for bank turns and landings.” Flaps on a space capsule could be a major advance over the long-standing use of bank-angle steering. Employed by space vehicles, including the Space Shuttle, bank-angle steering uses small thrusters to adjust the direction of lift generated by the vehicle. But more ambitious exploration missions may require more advanced control systems, such as flaps, to achieve their objectives.
Transporting soil samples back to Earth
Even as he plans for missions beyond the Feb. 18 Mars landing, Putnam’s work remains tethered to Perseverance. Outfitted with cameras, sensors, radar, spectrometers, microphones, aluminum wheels with terrain-gripping cleats, a helicopter drone named Ingenuity and autonomous guidance, as well as 24/7 supervision beamed up from NASA, Perseverance is roving Mars with a prime mission to find evidence of life.
Identified through copious, detailed photographs relayed back by orbiting satellites, the Jezero crater is a 28-mile-wide hole near the Martian equator, hollowed out by the impact of a long-ago meteor or asteroid. It appears to have once been a lake, with rock layers as old as 3.6 billion years, and a formation that resembles the mouth of a river. If life once inhabited the former lake, soil and rock samples may yield fossil evidence, such as microbe biosignatures. Perseverance is programmed to troll the crater’s edge, drilling rock, collecting samples and packaging them in sterile steel tubes for later retrieval.
That retrieval ties in significantly to Putnam’s work on reentry. Dubbed with signature NASA terseness as a “sample return,” the next major mission in the U.S. campaign for the Red Planet goes like this. Land a spacecraft on Mars, which sends its own rover to collect the sample tubes left behind by Perseverance. Then—and here comes the crazy sky-crane part—launch the tubes into orbit where they will be scooped up and returned to Earth by another space vessel. That probably would take that heavier, “SUV-size” spacecraft to carry the equipment needed to retrieve the samples and fling them into space. And that would seem to entail losing the chute.
It also will take an infusion of several billion dollars into the efforts of the vast network of dedicated researchers and technicians working at government facilities, universities and research centers across the nation on a complex mass of interlocking technologies expected to come together in a sample return mission sometime around 2030.
Putnam’s piece of that mission—the piece that gets the next spacecraft safely through Mars’ atmosphere to the surface—is like a hologrammatic replication of the whole amazing effort, a small but critical component that is itself of stultifying complexity and uncertainty. In accounting for factors that range from ablation—the chemical reaction that enables the heat shield to dissipate thermal energy while keeping the aeroshell containing the rover safe behind it—to the hypersonic aerodynamics of a reentry vehicle traveling at Mach 25, Putnam relies on a range of aerodynamic research. He credits his colleagues at the Center for Hypersonics and Entry Systems Studies (CHESS), a new interdisciplinary research unit in the Grainger College of Engineering at Illinois, for research support.
Landing humans on Mars
Beginning with the launch that put Alan Shepard into space, nearly 60 years of very expensive, painfully deliberate and detailed, edge-of-the envelope-risky R&D has been driven by the desire to know what’s out there. NASA has been setting spacecraft down on Mars since July 20, 1976, when Viking 1 sailed down to the planet on a parachute not all that different from the one deployed by Perseverance on Feb. 18, 2021. The U.S. now has three orbiters circling Mars, with the European Space Agency having two and the Indian Space Research Organisation having one. They all regularly send back data about the planet’s atmosphere and geology, which will have helped pinpoint the Perseverance’s landing time and place. The rover’s older sibling, Curiosity, still roams Mars collecting data about the planet’s biology, geology, chemistry, atmosphere and radiation.
Still, another half-century could intervene before people alight on the Red Planet. With the Perseverance weighing 2,204 pounds, or a metric ton, and the sample return craft weighing possibly twice that, Putnam estimates that the minimum size for a human-class lander would be 20 metric tons. For a crew to land on Mars, live there and explore, and get back to Earth, a payload of 100 metric tons might be more realistic. So, instead of bringing everything in one enormous ship, Putnam says the bulk of the mission’s needs could be divided among several 20-ton spacecraft piloted to Mars by robots. This would allow living spaces, supplies and a return rocket to be prepositioned. “All of those things [would be] sitting there waiting for them when they show up,” Putnam says. “And those earlier landers would essentially be robotic.”
Meanwhile, Hollywood has ignored the parachute problem and made it to Mars. Lots of times. Stars like Matt Damon in The Martian and Hilary Swank in Away have ridden technology-enabled sci-fi scripts to the planet that gleams, so red and tantalizing, so easy to see but upwards of 33 million miles out of reach. The space travel, the landings, the adaptable technology, the mastery of Mars seem inevitable, at least on screen. As for Zach Putnam, he’s cheerfully immersed in the synapse-frying task of getting a real crew to the Red Planet. It will be an achievement second to none for America and the human race. “It would be great,” he says, smiling, “to see someone set foot on Mars in my lifetime.”
SCI-FI INVASION
What we see when we see mars

English Professor Robert Markley’s book, Dying Planet, surveys the evolution of Mars-inspired literature and film. (Duke University Press)
Ever since people started looking up at the night sky, the Red Planet has pulsed with wonder, fear and reflection. “We’ve long been fascinated by Mars because it looks in many ways like an analog for Earth,” observes Robert Markley, a U of I English professor who melds 18th-century literature, planetary science and science fiction to write about what we see when we see Mars.
Markley’s 2005 book, Dying Planet, covers the evolution of Mars-inspired literature and film. Toward the end of the Victorian era, astronomers’ observations of the planet unleashed a flood of imaginative literature, including The War of the Worlds by H.G. Wells. That book, with its terrifying force of Martian occupation, marched on into 20th-century films, television shows, comic books, video games, and of course, Orson Welles’ notorious radio play. During the 1960s, Markley notes, “About 75 percent of the science fiction published in the U.S., Britain and Australia featured Mars in a prominent role.”
More recently, Kim Stanley Robinson’s Mars trilogy presents a sweeping sci-fi panorama of life on the Red Planet. Published in the 1990s, the novels envision colonization of Mars through “terraforming”—an imagined process that makes other worlds livable by “creating a greenhouse effect, warming the atmosphere so it can hold more water vapor,” explains Markley, who published a book on Robinson in 2019. Far-fetched as the idea might sound, terraforming has received serious attention from scientists, including the late Carl Sagan.
As for the real Mars, NASA orbiters send back forbidding tales of its barren surface, carbon-dioxide atmosphere and sub-sub-zero temperatures. But the data also reveal a planet that is geologically diverse. “If we could go back and stand on the Martian surface 3.75 billion years ago, it would look like a very weird Earth-like planet with water,” Markley concludes. “And who knows what else might be there?”
What’s certain is that Mars will always claim our attention—33 million miles away, but as close as a space landing, a good read or a telescope in the backyard.—M.T.
VITAL SIGNS
Could a microbe found on Earth also be present on Mars?
For geobiologist Bruce Fouke, the rocks collected on Mars by the Perseverance rover may answer a question that has surfaced throughout his career: Could life on another planet be like life on Earth?
Fouke, a U of I professor who studies fossil traces of microbial organisms, believes a microben organism found in Yellowstone National Park might also have inhabited the Red Planet. Dubbed Sulfurihydrogenibium yellowstonense—“Sulfuri for short,” he says, grinning—the microbe lives underground, surfacing in Yellowstone’s iconic hot springs and leaving its biosignature behind in long strands of calcium carbonate that Fouke delights in comparing to fettuccine. In 2019, he led a NASA-funded study to analyze the tough little life form’s genome.
Beyond investigating microbes in Yellowstone and other settings around the globe, Fouke studies fragments of asteroids and meteors for signs of entombed microbial life. But rocks from outer space require even more extensive study to establish their origins and how long they’ve been on Earth. Fouke is eager to see what comes back from Mars. “Nothing,” he says, “can replace a pristine sample.”
The Perseverance will retrieve rocks and soil from the planet’s Jezero Crater—an area that appears to have once had its own hot springs. “The hairs on my neck stand up as we’re talking about it,” Fouke says. With those samples expected to arrive no sooner than 2030, though, he adds, with charming irony, “They will probably get here from Mars right about the time I decide I’m going to retire.”—MT

Geobiologist Bruce Fouke collects samples from Mammoth Hot Springs in Yellowstone National Park. (Tom Murphy)