Famine Fighters
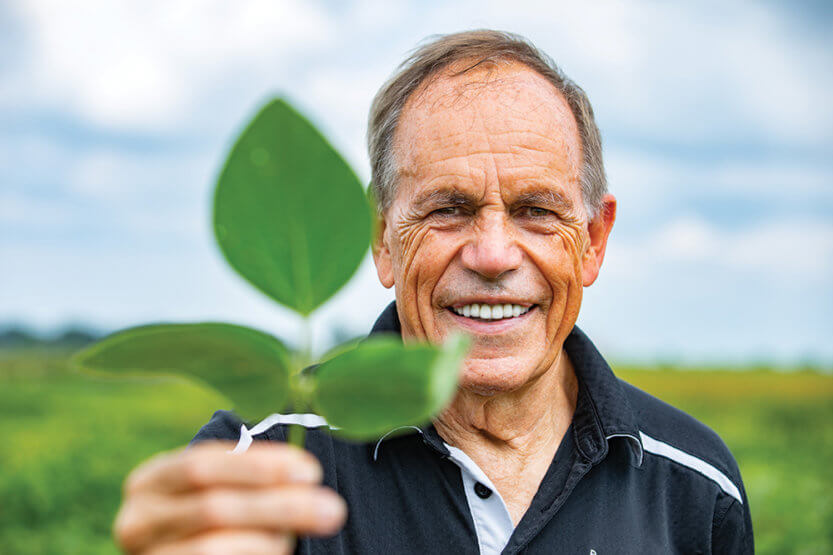
It’s high noon on an unforgiving August day, storm clouds blotting out the bright blue sky, the sun a source of malice and spite. Steve Long, British-born and a boyish 71, takes the Illinois heat in stride—an occupational hazard—as he walks through the 10-acre field, soybeans stretching as far as the eye can see. Though the morning rain has mostly burned away, the smell of humus is still in the air, and the soybeans’ freshly watered leaves are a deep green that meet Long’s approval, as he makes his rounds. But these are no ordinary soybeans—this one field contains more than 600 different varieties—and Long is no ordinary farmer.
A U of I professor of crop sciences and plant biology, Long is one of the planet’s leading researchers on photosynthesis—the process through which plants transform sunlight into energy. For more than 20 years, he and his Illinois colleagues have been expanding science’s understanding of how that process works, one research study at a time. Now, they’re using everything they’ve learned to confront one of Earth’s greatest challenges—world hunger.
If their plan is successful, they will re-write the future of humanity itself.
AVERTING DISASTER
From continent to continent and culture to culture, famine has been an unavoidable fact of the human experience for all of recorded history. But in recent decades, with climate change altering our growing seasons and the global population increasing exponentially, the problem has taken on a sense of urgency.
“Today, there are close to a billion people who are calorie insufficient,” says Long, mopping his brow amid the summer heat. “Since 2014, that number has been rising.”
And it’s only going to get worse. As the population continues to grow, the amount of arable land and natural resources will decrease. By 2050, the projected global population will be 9.7 billion—nearly 2 billion more than today. According to the United Nations’ Food and Agriculture Organization, food production will have to increase by 70 percent to meet that demand, and potentially more, depending on the impact climate change will have on crop yields.
In response, Long and his colleagues created RIPE (Realizing Increased Photosynthetic Efficiency), an ambitious, international research program that is attempting to redesign the future of food production.
How? By using genetic engineering to dramatically increase yields of staple crops in regions that will be most affected by population growth.
Under RIPE’s plan, small farmers in sub-Saharan Africa and southeast Asia will grow those new crops (including soybeans, maize, cassava, cow-pea and rice), raise enough food for their families and for the market, and avert disaster, saving millions of lives.
COMPUTER SCIENCE MEETS AG SCIENCE
RIPE’s origins go back to the early 2000s—the Dark Ages of computer modeling—when Long and his colleagues became the first scientists to simulate the process of photosynthesis in a leaf.
The experiment was something Long had wanted to do for years, and now that he was a professor at Illinois, a university with supercomputing power, he could finally do it. He was excited, his collaborator Don Ort was excited, they ran the experiment, and then, to their surprise, they made a discovery: photosynthesis, the process that has produced all the oxygen in our atmosphere and serves as the main conduit for carbon dioxide into plants, doesn’t always work.
In fact, they found that it’s pretty inefficient: it fails 20 to 25 percent of the time—when the RuBisCo enzyme, the most abundant protein on Earth, mistakenly reacts with oxygen instead of carbon dioxide—and even when the process works perfectly, the plant still only uses 3 percent of all the light energy it receives.
Those discoveries led the researchers to ask: Is it possible to redesign the process of photosynthesis to make it more efficient? In other words, to give it an upgrade?
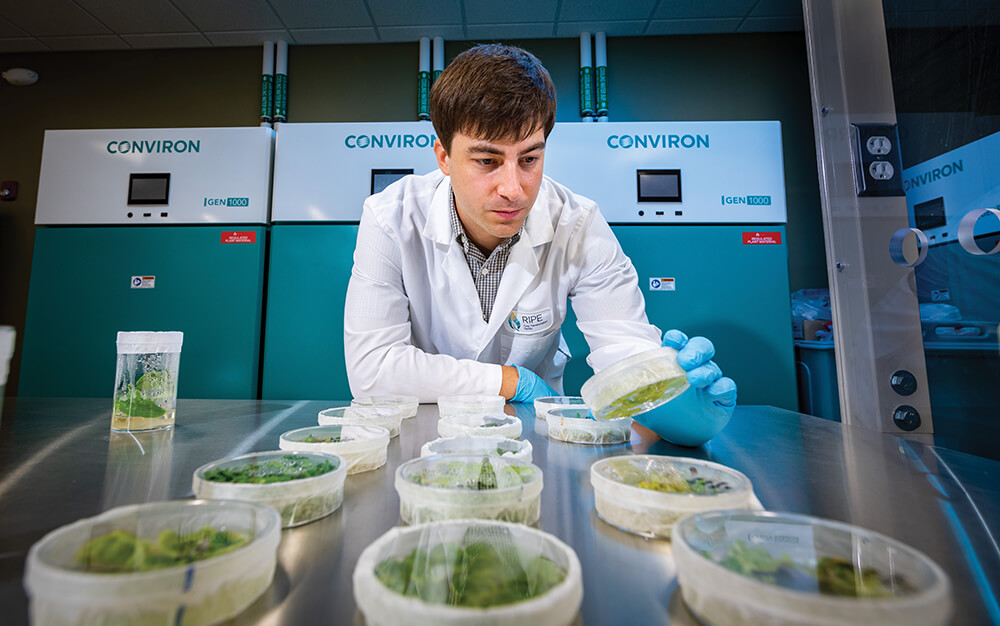
“Failure is a big part of laboratory research, so one has to be very resilient to that,” says Benjamin Haas, who oversees RIPE’s DNA assembly work on campus. (Image by Fred Zwicky)
In a series of groundbreaking studies, they determined that, in theory, it was possible. And what’s more, the upgrade could help save humanity from catastrophe. More efficient photosynthesis would not only lead to bigger plants, but also cause those plants to absorb greater amounts of carbon dioxide from the atmosphere. The result: a dramatic increase in crop yields and a method to address climate change.
Those findings got the attention of the scientific community—and perhaps more importantly, they got the attention of those who fund the scientific community.
In 2012, Long received the sort of call that most scientists dream of but know will never come: the Bill and Melinda Gates Foundation had read his team’s research and believed that it could be a direct solution to world hunger. They were willing to fund an unprecedented, not-for-profit, international research project to see if it would work.
Ten years later, RIPE has received more than $83 million in funding, every dollar getting them closer and closer to their goal of redesigning photosynthesis.
ENHANCED PHOTOSYNTHESIS
The RIPE Plant Transformation Facility is a nondescript, brick-and-stone building on the southern edge of the Illinois campus. Inside, biotechnologists wearing long, white coats use a variety of methods to transform model plants for their experiments: prediction-based cloning, Golden Gate Assembly, loop assembly—concepts that sound vaguely futuristic but are nonetheless hallmarks of genetic engineering the world over.
Look around the lab and you’ll see familiar sights, even if your last science class was in high school: beakers, pipettes, bottles, scales, washing stations, refrigerators and WARNING! DANGER! signs. But one of the most familiar things you’ll see will also be the most surprising: a series of clear, plastic, domed coffee cups, the kind that usually contain Frappuccinos. Only these cups have nothing to do with coffee—each one is a miniature greenhouse for a tobacco plant.
Yes, that tobacco—one of the most contemptible plants in the history of the Earth. But for RIPE’s scientists, tobacco is something else entirely: the gateway plant for redesigning photosynthesis. Why? Simply put, “it’s very easy to transform, and it produces a lot of seed,” says Long.
Not only that, but because tobacco grows quickly—eight weeks from seed to mature plant—researchers are able to run more rounds of experiments on it than other potential subjects. “It’s a really great test for our hypotheses,” says Benjamin Haas, who oversees the project’s DNA assembly work on campus.
Those hypotheses come not only from the RIPE team at Illinois, but also from their research partners around the world—in the U.S., the U.K., Australia and China—all of which have specific expertise related to plant biology and genetics, and highly defined roles within the project.
“We’re trying to improve a process that has been around for a very long time but developed in an environment that was much different from the Earth today,” says Haas. “It’s a complicated process, and that’s why we have so many researchers working on very specific angles of it.”
Whenever researchers believe they’ve found something promising, they send it to the Plant Transformation Facility. There, day by day, Haas and his colleagues test the viability of hypotheses, DNA vectors and genetic constructs, all through the magic of bioengineering.
The appeal of this approach, for Haas, is that it allows him to insert a “super-tiny” portion of a genome from one organism into another, with a level of precision that was impossible only a few decades ago, when conventional plant breeding was the norm. “You actually know what the gene you’re introducing does, or at least what it should do,” Haas says, “and you’re able to test how it impacts the plant as it grows.”
Inside the coffee cup greenhouses, Haas and his colleagues grow tobacco from tissue cultures, imbued with genetic material from pumpkins and algae, the genomes they’re currently testing. Each cup contains a specially made gel at the bottom, which encourages root and shoot development, and soon, with light and water and air, the plant begins to grow.
Before the plants fill the cups, Haas and Co. carefully wash the gel from the roots, transfer the plants into small pots and move them into an incubator, which helps to stabilize their growth before they reach their next stop: a RIPE greenhouse.
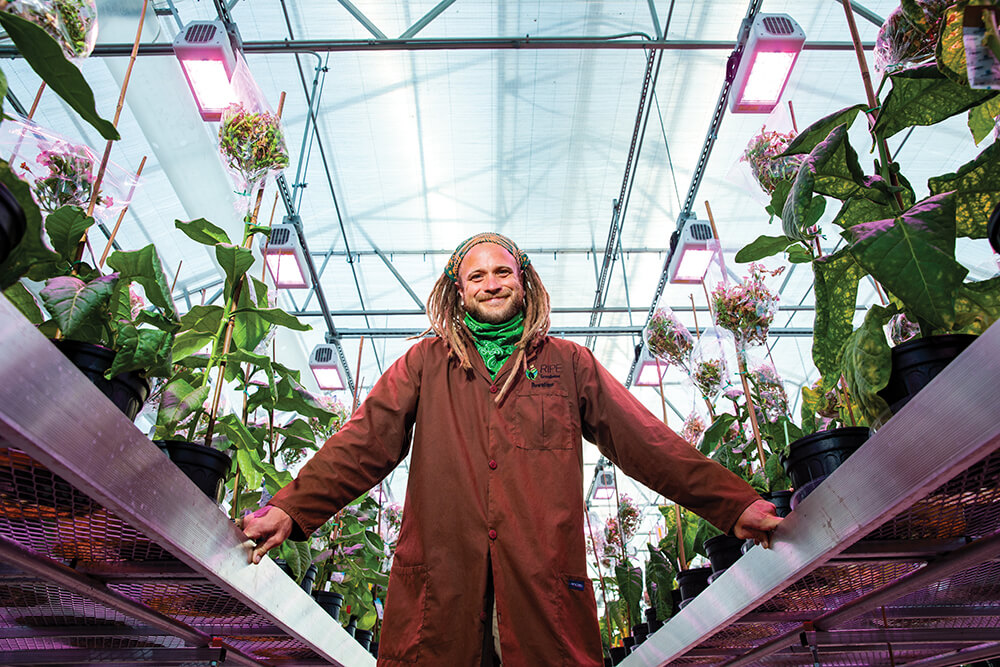
“Everything about this place is a step above what we were doing before,” says David Drag about RIPE’s state-of-the-art greenhouse. (Image by Fred Zwicky)
BRIGHTER LEAVES
Across the street from the Plant Transformation Lab, inside the High-Throughput Phenotyping Facility, David Drag, ’08 LAS, MS ’14 LAS, administers RIPE’s state-of-the-art greenhouse: a long, open room with diaphanous windows as walls, the translucent ceiling so high you couldn’t reach it with a Super Ball. Down the center, stainless steel benches host row upon row of mature tobacco plants, their bright-pink flowers one of the few bits of splash in an otherwise muted space.
Overhead, a series of high- powered LEDs provides the amount of light the plants receive. From an app on his phone, Drag can adjust the LEDs, one-by-one or in groups, making them as bright or dim as needed.
“You can’t beat the spectrum on these,” he says. “With more traditional lighting, you just don’t see such a deep green color in the leaves. You also notice a difference in the thickness of the leaf texture.”
The lighting is only one thing that makes this greenhouse feel unique. It also has acrylic double walls that hold in air and prevent heat loss; a humidification system that keeps the relative humidity between a minimum of 50 and 60 percent at all times; and geothermal wells that maintain the temperature and eliminate the need for outside vents, which prevents insect- and pest-infestation and ensures an ideal environment for the plants.
Finally, the crème de la crème of the whole operation: the Lidar system. Lidar, an acronym for “light detection and ranging,” is a 3D scanning technology that allows RIPE’s researchers to collect data about every aspect of a plant’s growth and maturation. Lidar’s multispectral cameras move around the greenhouse on a crane system, all the while creating a fully formed digital image of a plant that can be rotated 360 degrees in any direction. In only 90 minutes—the scanning time of the machine—Lidar is able to collect precise data about the dimensions, greenness and other physical qualities from 408 plants, each labeled with a barcode. “It’s incredible,” says Drag. “Using traditional measurements, it would take us over an hour to get that data for a single plant!”
Below the Lidar system, stretching more than half the length of the greenhouse, four rows of gray, industrial-looking scales sit on the floor, waiting for their next round of plants. “They’ll be grown there from start to finish,” says Drag, so that the scales can provide real-time monitoring of each plant’s weight, deploy their built-in irrigation system as necessary, and measure the plant’s water use and biomass over the course of its growth.
At the end of each experiment, RIPE uses all the data they’ve collected from Lidar and the scales to determine whether the plants demonstrated an improvement in photosynthetic efficiency.
If the experiment fails, Haas and his colleagues analyze the data to determine what happened, then they make adjustments, insert new genes and try again. Meanwhile, Drag prepares for the next round of plants from across the street.
But if the experiment succeeds, and the tobacco plants demonstrate an increase in photosynthetic efficiency, Haas and Co. use E. coli bacteria to replicate the DNA from those plants in large quantities. From there, they isolate the DNA and introduce a natural soil pathogen as a vector, to insert the successful genes into soybean plants. Finally, they repeat the experiment with the soybeans and hope for the best.
If the experiment succeeds in soybeans, the next round of that genotype will graduate to RIPE’s true testing ground: the field trial.
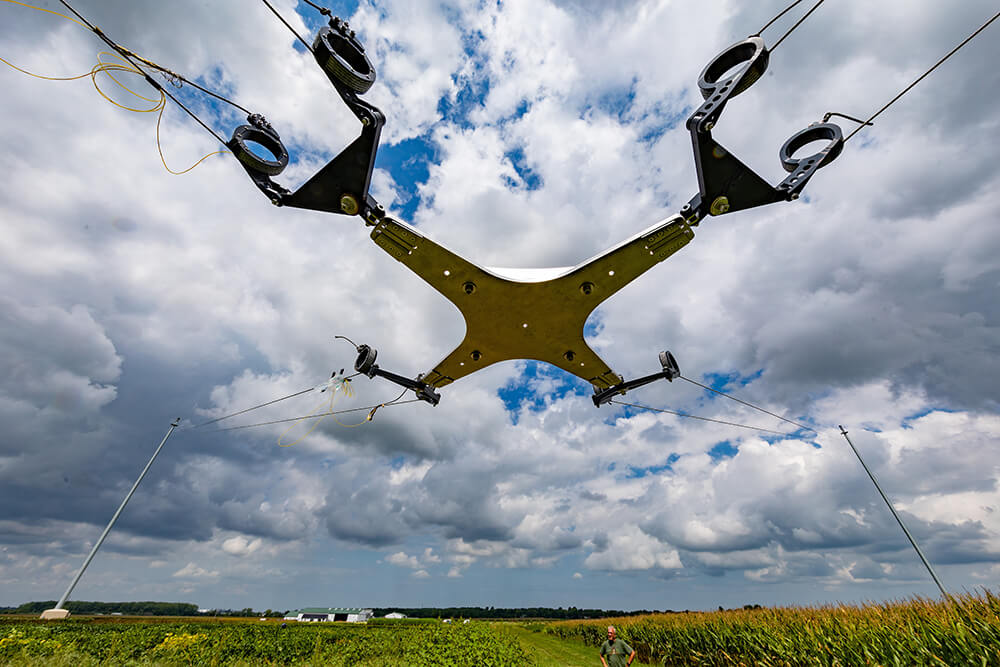
“We’ve seen hawks perched at the tops of those towers,” says Brett Feddersen (shown in background) about the massive pillars that hold RIPE’s spider cam imaging system in place. “It must be a good hunting spot.” (Image by Fred Zwicky)
FIELD-TESTED
A mile south of Urbana are the RIPE project fields, which from the road look like legions of other fields, on farms all over central Illinois. But a closer examination yields a few key differences.
For one thing, the soybeans aren’t planted in uniform rows, like you’d see in your Uncle Alan’s field back home. Instead, they seem to be planted in distinct groupings, as if they’re not all of the same variety (and they’re not). Some are covered with red and silver aluminum foil, their carbon dioxide and water use monitored by machines that look like robots from the Pixar movie WALL-E. Others seem to be left to their own devices, taking in water and sunshine like any other plant on a summer day.
Not far from the robots, in a clearing between fields, a large canvas tent gives shade to a team of crop scientists, as they clip and label thousands of soybean plants fresh from the ground—testing their color, size, seed pods and other attributes. Nearby, a long white trailer houses offices and computers. And above it all, suspended high in the sky, is the biggest agricultural camera array on Earth. Imagine a bird’s eye scanner at your local library, but one that’s connected to high-tension pulleys and is large enough to cover an entire 10-acre field. That is the spider cam, a system that uses the same technology as stadium cams at sporting events. Unlike the stadium cam, however, this type of camera is capable of collecting biological data—in fact, the same data Drag collects in the greenhouse, but in a field setting.
From his modest command center in the on-site trailer, Senior Research Programmer Brett Feddersen, MS ’86 ENG, PHD ’93 ENG, MBA ’99, controls the location and altitude of the array, scans hundreds of crops in a matter of minutes, and collects and analyzes data that would have been unobtainable only a few years ago.
He and his colleagues use that data to determine whether the plants that were successful in the greenhouse can withstand the changing environment of the great outdoors. For Drag, the project’s field trials manager, that’s the moment
of truth.
“We’re trying to make a more productive plant that farmers already know how to grow, and the field trial captures that chaos of growing outside,” Drag says. “Weather is our worst enemy, and we need to make sure these plants are going to survive the way we want them to, under all sorts of conditions, so they’ll increase yields and feed more people.”
REGULATORY HURDLES
If all goes well, and RIPE does find a consistent solution for redesigning photosynthesis, it will still face an uphill battle to get its crops to the farmers who need them.
That’s because the crops in RIPE’s experiments are not merely plants in a field: they’re transgenic, or genetically modified, organisms (GMOs) that will need to pass test after test, and meet a laundry list of government regulations before they can be made available to consumers.
According to U.S. Dept. of Agriculture rules, if RIPE’s crops are successful in their field trials, they will then need to undergo multi-location field trials elsewhere, a process that often takes up to five years. From there, the Food and Drug Administration will require another 10 years of safety testing to make sure the crops are suitable for human consumption and that they won’t harm local ecologies in their growing areas.
Regulations over transgenic crops were first introduced in the late 1990s, after environmental groups launched aggressive campaigns against GMOs and the agribusiness corporations that created them. They were concerned that not enough research had been conducted on these new plants and that their consumption might be unsafe for humans; that the most popular genetically modified crops, such as Monsanto’s “Roundup Ready” corn and soybeans, encouraged the rampant use of cancer-causing pesticides; and that the rapidly growing popularity of those crops would lead to monoculture farming, which would ruin agricultural diversity.
In the media firestorm that followed, public sentiment toward GMOs soured in the U.S. and around the world. Governments responded with strict GMO regulations, and consumer interest in organic, non-GMO foods began a slow growth that has exploded over the past decade. (According to The New York Times, nearly half of today’s U.S. shoppers try to avoid genetically modified foods.)
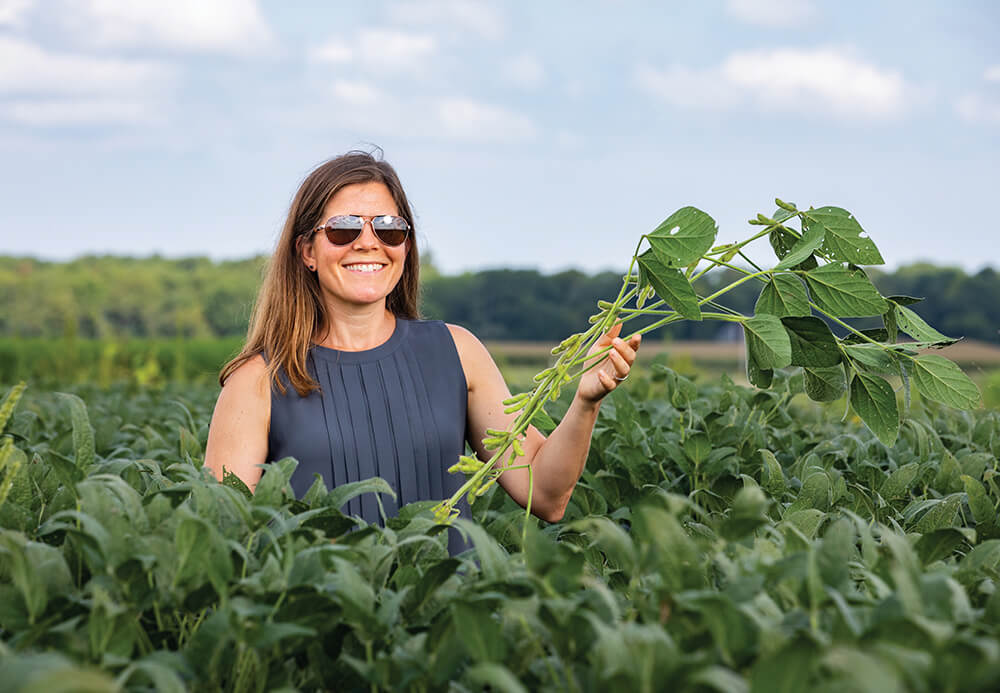
“When I was a graduate student at Illinois, I took ‘Plants and Global Change’ with Steve Long and ‘Photosynthesis’ with Don Ort. Then later, I taught ‘Photosynthesis’ to David Drag. And now we all work together,” says Lisa Ainsworth, RIPE’s deputy director. “I think one of the things Steve and Don do brilliantly is build teams that are more like families and really buy in to what they’re doing.” (Image by Fred Zwicky)
“We never really had an honest conversation about genetically modified organisms in this country before they were 90 percent of the soybeans and 85 percent of the cornfields,” says Lisa Ainsworth, PHD ’03 ACES, RIPE’s deputy director. “We need to have those conversations, to explain to people that the technology we’re using is essentially the same technology that’s used to make all sorts of stuff, from insulin to COVID vaccines. Scientists haven’t done a good job of talking about the technology and it’s been confusing to the public. And a lot of our success will come from how we frame the conversation and educate people.”
In recent years, many environmental groups have changed their minds about GMOs, in response to long-term research studies, which have proven that transgenics are safe to eat and are not harmful to the environment.
But public sentiment, formed and hardened nearly a quarter-century ago, has remained largely negative. And the necessary, but perhaps exceedingly strict, government regulations remain in place.
“From start to finish, it could take anywhere from 15 to 20 years to get these crops to farmers,” says Drag. And that’s if—a big if—the crops don’t face additional resistance from governments, activists and farmers in Africa and Asia, where GMOs are even more demonized than they are in the U.S.
Despite the lengthy timeline and the high potential for anti-GMO backlash, Long believes that RIPE’s approach is the correct one—the plan that has the greatest capacity to increase food security for the greatest number of people.
When Long and his colleagues first began talking about this project with the Gates Foundation more than a decade ago, one of Bill Gates’ advisors voiced concerns about the controversy over transgenic crops. According to Long, Gates said, “Yes, but if we do nothing now, and in 20 years’ time we finally need this, it’s going to be too late. We need to insure against the future.”
“The world is going to solve its population growth problems,” Long says. “But it would be best if we could avoid doing that through starvation. And that is what RIPE is trying to do.”